
Understanding Dominant Traits
Definition of Traits
A trait is an attribute or characteristic that makes an individual unique. It can refer to physical characteristics influenced by genetics, such as having green eyes or a specific height. On a broader level, traits can also encompass aspects of an individual's personality or behavior. Understanding traits is essential for exploring how certain characteristics can express themselves.
The Concept of Dominant Traits
Dominant traits are specific characteristics that require only one copy of a dominant allele to be expressed. In genetics, an allele is a version of a gene, and a dominant allele can mask the influence of a recessive allele. Therefore, even if an individual has one dominant allele paired with one recessive allele, the dominant trait will be the one that manifests.
For a clearer view, consider the following characteristics of dominant and recessive traits:
Trait TypeAllele RequirementExpressionDominant1 copyExpressed regardless of the second alleleRecessive2 copiesExpressed only if both alleles are recessive
In practical terms, if an individual inherits one pathogenic dominant allele related to a specific trait, they will express that dominant trait. Dominant traits are typically inherited vertically from parent to child, affecting both genders equally. Each child has a 50% chance of inheriting the trait from a parent carrying the dominant allele [2].
An example of a trait demonstrating both dominant and recessive patterns is the sickle-cell allele. It requires two copies to induce sickle-cell disease but provides malaria resistance with just one copy [2].
Understanding dominant traits is crucial for exploring how traits can potentially mask others, enriching the discussion on various genetic conditions like autism. For more insights on autism, consider visiting our sections on autism types and symptoms of mild autism.
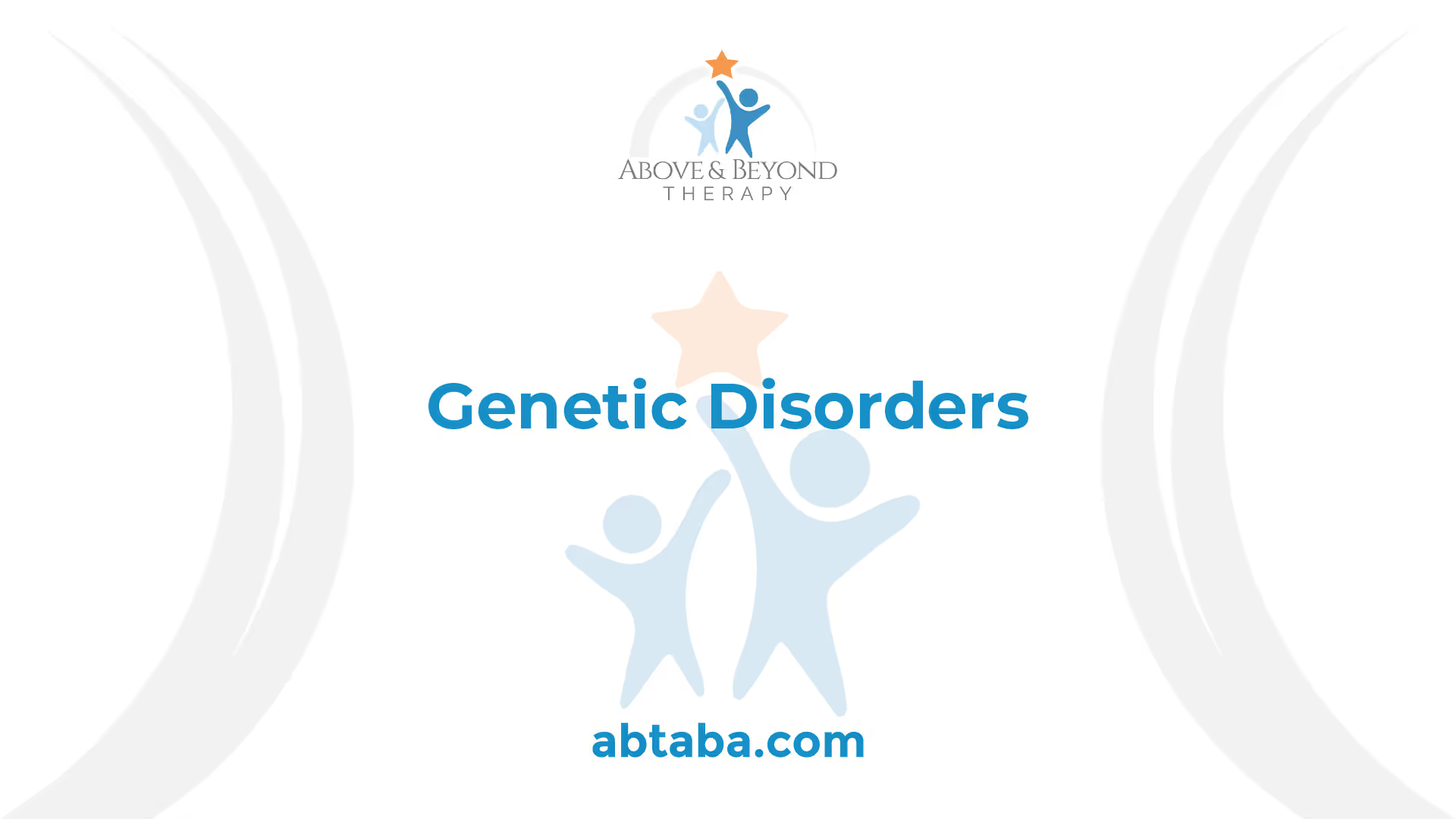
Genetics of Dominance
Understanding how traits are inherited is crucial in genetics. In this section, the focus will be on dominant and recessive alleles, which play vital roles in determining physical characteristics, including various traits.
Dominant Alleles
A dominant allele is defined as an allele that produces a dominant phenotype in individuals possessing just one copy of that allele. This means that even if only one dominant allele is present, the trait associated with it will be expressed. The specific allele that can mask another is known as a dominant trait. For example, if an individual inherits a dominant allele for brown eyes and a recessive allele for blue eyes, the person will exhibit brown eyes due to the dominance of the brown eye allele.
The table below summarizes the characteristics of dominant alleles:
Allele TypeRequired Copies for ExpressionEffect on Recessive AlleleDominant Allele1Masks the effect
Recessive Alleles
Conversely, recessive alleles require two copies in an individual to manifest as a phenotype. This means that an individual must inherit recessive alleles from both parents for the trait to be expressed. If an individual has one dominant allele and one recessive allele, the effect of the recessive allele is masked by the dominant allele, resulting in the expression of the dominant trait.
Here’s a table highlighting the characteristics of recessive alleles:
Allele TypeRequired Copies for ExpressionEffect on Dominant AlleleRecessive Allele2Remains unexpressed
In genetics, understanding these principles is key for exploring more complex interactions and traits. For further insights into variations of traits and conditions, one can explore topics such as autism types or the different symptoms of mild autism.

Examples in Genetics
Understanding dominant traits is crucial in genetics, and historical experiments help illustrate these concepts clearly. Key examples include Gregor Mendel's pea plant experiments and the study of dihybrid crosses.
Mendel's Pea Plant Experiments
Gregor Mendel, often known as the father of genetics, conducted experiments on pea plants to study inheritance patterns. He discovered that when pure-breeding pea plants with different traits were crossed, the resulting offspring (F1 generation) displayed only one of the parental traits, exemplifying dominance.
In a particular study, when two pea plants with pure white flowers were crossed, the F2 generation exhibited a phenotypic ratio of approximately 9:7, a result that deviated from standard Mendelian ratios. This outcome highlighted a trait that could potentially mask the effects of another gene's alleles, emphasizing the complexity of genetic inheritance.
Parental TraitsF1 Generation TraitsF2 Generation RatioPure White Flowers (aa) x Pure Purple Flowers (AA)All Purple Flowers (Aa)9:7
Researchers identified two genes that contribute to this 9:7 ratio, controlling flower color by regulating biochemical pathways related to pigment compounds. The presence of specific alleles determined the outcome of flower color.
Dihybrid Crosses
Dihybrid crosses involve two genes instead of one, allowing geneticists to observe interactions between multiple traits. In these crosses, the phenotypic ratio can differ significantly from the expected 9:3:3:1 ratio seen in monohybrid crosses.
For instance, in a dihybrid cross of pea plants, researchers observed that specific traits displayed complex interactions, often resulting from dominant suppression or epistasis. In such cases, one trait can mask the expression of another, modifying the classic Mendelian ratios.
Trait PairExpected RatioObserved RatioSeed Shape and Color9:3:3:1Deviated Ratio Due to Interactions
These principles help contextualize how certain traits can mask others, a concept that can also be seen in various genetic conditions, including autism. Understanding these interactions sheds light on the complexities of gene expression and inheritance. For more on traits related to autism, visit our sections on autism types, forms of autism, and autism scale.
Types of Gene Interactions
Gene interactions play a crucial role in how traits are expressed and can result in one trait overshadowing or modifying another. This phenomenon is often seen in cases where a trait that can possibly mask another trait is known as a trait. In this section, we will discuss two types of gene interactions: epistasis and dominant suppression.
Epistasis
Epistasis occurs when the effects of one gene can mask or modify the expression of another gene, leading to a complex interaction between different alleles. This can result in observed phenotypes that deviate from expected Mendelian ratios.
A notable example of epistasis is seen in the study of flower color in peas conducted by Bateson and Punnett. In their experiments, one "allelomorphic pair" was able to mask the effects of alleles for another gene, leading to unexpected ratios in flower coloration. Additionally, research on wheat kernel color demonstrated how the presence of specific alleles determined the kernel color outcomes, depending on biochemical reactions involving multiple genes.
In cases like the horse coat color example, interaction among genes can show how one gene might influence the expression of another without fully masking it. The extension gene determines basic colors, while a modifier gene might alter the pigment shade [3].
Dominant Suppression
Dominant suppression refers to a situation wherein the presence of a dominant allele can completely suppress the expression of another allele. This means that even if the recessive allele is present, it may not manifest in the phenotype. This concept is closely related to dominant traits, where the dominant allele’s effect is stronger and visible, while the recessive allele remains hidden.
One relevant example involves the sickle-cell allele, which illustrates how a trait can exhibit both dominant and recessive patterns. Although sickle-cell disease itself follows a recessive inheritance pattern and requires two copies for the disease to manifest, the same allele offers dominant protection against malaria when one copy is present [2].
Understanding these interactions is essential in genetics, especially when studying conditions where traits can mask others. This knowledge can contribute greatly to the field, including the study of conditions such as autistic disorder where genetic factors may play a significant role in manifestation and behavior patterns.
Genetic Disorders
Genetic disorders can arise from mutations in specific genes, leading to various health implications. This section will focus on two notable genetic disorders: Noonan Syndrome and Arrhythmogenic Right Ventricular Dysplasia/Cardiomyopathy (ARVD/C).
Noonan Syndrome
Noonan Syndrome is a genetic condition that results from mutations in various genes, including PTPN11, SOS1, KRAS, RAF1, BRAF, MEK1, and more recently, SHOC2. These mutations lead to upregulated RAS-MAPK signaling, which occurs due to reduced GTPase activity or increased guanine nucleotide release. This disruption causes abnormal signaling through the MAPK cascade, impacting numerous developmental processes of the body [4].
Individuals with Noonan Syndrome may exhibit a variety of symptoms, including distinct facial features, short stature, and congenital heart defects. The condition is often diagnosed through genetic testing, which can identify the specific mutations responsible.
Symptoms of Noonan SyndromeDistinctive facial featuresShort statureCongenital heart defectsBleeding disordersDevelopmental delays
About 95% of cases of a related condition, LEOPARD syndrome, are due to mutations in the PTPN11, BRAF, and RAF1 genes. This syndrome includes symptoms like multiple lentigines, café-au-lait spots, and a higher prevalence of electrocardiographic conduction abnormalities [4].
Arrhythmogenic Right Ventricular Dysplasia/Cardiomyopathy (ARVD/C)
Arrhythmogenic Right Ventricular Dysplasia/Cardiomyopathy (ARVD/C) is another genetic disorder characterized by the replacement of heart muscle with fibrous or fatty tissue. This condition primarily affects the right ventricle and can lead to serious heart issues, including arrhythmias and sudden cardiac arrest.
ARVD/C is often inherited in an autosomal dominant manner, which means that only one copy of the mutated gene is needed to manifest the disease. The disorder is frequently caused by mutations in genes associated with desmosomes, which are crucial for cell adhesion in heart muscle cells.
Symptoms of ARVD/CPalpitationsLight-headedness or dizzinessFainting (syncope)Heart failure symptomsSudden cardiac arrest
Though ARVD/C symptoms may vary among individuals, chest pain, palpitations, and syncope are common signs. Diagnosis typically involves a combination of family history assessment, imaging studies, and genetic testing.
Understanding these genetic disorders is crucial for research and the development of potential treatment strategies. For those interested in autism and its variances, additional insights can be found in our articles about autism types and forms of autism.
References
[2]:
[3]:
[4]: